Illustration of Aging Effects on Cellular and ECM Structures
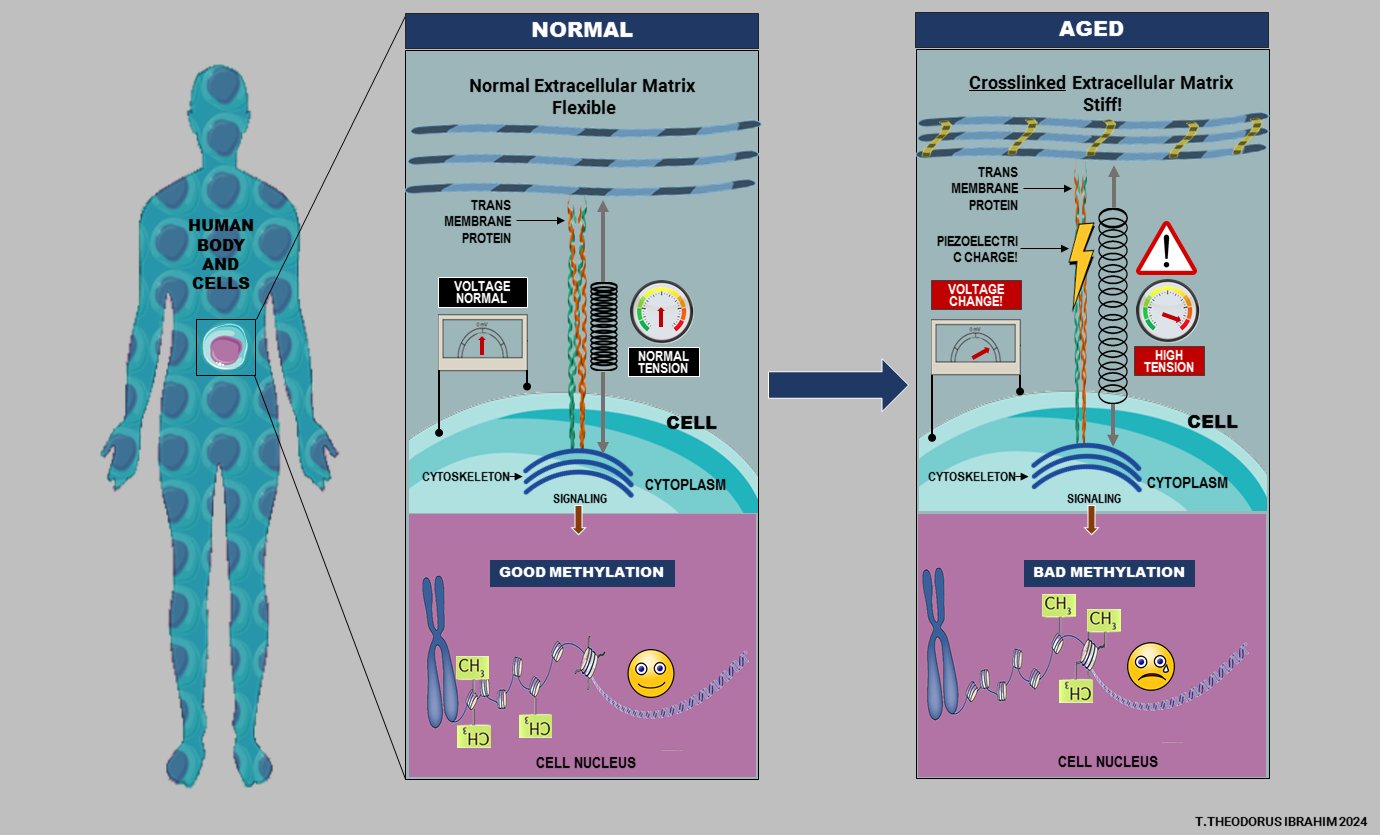
This image illustrates the effects of aging on cellular and extracellular structures, focusing on changes in the extracellular matrix (ECM), cellular voltage, and methylation patterns.
Description and Analysis
Normal State (Left Panel):
- Flexible Extracellular Matrix: In the normal state, the ECM is flexible, allowing cells to communicate and function effectively.
- Transmembrane Proteins: These proteins connect the ECM with the cell, transmitting signals that maintain normal cellular function.
- Normal Voltage and Tension: A stable voltage reading and balanced cytoskeletal tension indicate a healthy, responsive cell environment.
- Good Methylation: Methylation patterns in DNA within the nucleus are well-regulated, supporting proper gene expression, cellular differentiation, and stability.
Aged State (Right Panel):
- Crosslinked, Stiff ECM: With aging, the ECM becomes more crosslinked and stiff, impairing flexibility and affecting cellular communication.
- Piezoelectric Charge: A buildup of piezoelectric charges can occur due to the increased stiffness, potentially disrupting cellular function.
- Voltage Change and High Tension: The increased stiffness leads to higher cytoskeletal tension and altered cell voltage, which could disrupt intracellular signaling.
- Bad Methylation: This state is associated with aberrant methylation patterns, which could lead to improper gene expression, accelerated aging, and health decline.
Implications
Bioelectric Health and Aging:
This diagram highlights how bioelectric health is influenced by the physical properties of cellular and extracellular environments. As cells experience increased mechanical and electrical stress due to ECM changes, they may undergo epigenetic alterations (such as aberrant methylation) that could accelerate aging and promote disease.
Relevance to ceLLM Theory:
This image resonates with concepts in ceLLM theory, as it suggests that changes in the extracellular matrix’s physical structure can influence cellular behavior and gene expression through electrical and mechanical pathways. Resonant and field-based interactions between cellular components may also play a role in how cells adapt (or fail to adapt) to environmental changes over time.
Detailed Mechanism: Piezoelectric Transmembrane Proteins and ECM Stiffness
The core mechanism involves the interaction between ECM stiffness and piezoelectric transmembrane proteins, which has profound effects on cellular voltage (Vmem) and gene expression within the ceLLM framework. Here’s how the process unfolds:
- Piezoelectric Transmembrane Proteins:
- Function: These proteins generate electrical charges in response to mechanical stress due to their piezoelectric properties.
- Role in Normal State: Under normal ECM flexibility, these proteins maintain a balanced Vmem, facilitating proper cellular signaling and function.
- ECM Stiffening with Aging:
- Cause: Aging leads to glycation and crosslinking of ECM components, resulting in increased stiffness.
- Effect on Proteins: The stiffer ECM exerts greater mechanical stress on transmembrane proteins.
- Charge Development and Vmem Alteration:
- Mechanism: The mechanical stress induces the piezoelectric transmembrane proteins to develop additional charges.
- Impact on Vmem: These new charges can either add to or subtract from the normal Vmem, disrupting the cell’s electrical balance.
- Disruption of ceLLM Governing Gene Expression:
- Faulty Inputs: Altered Vmem provides faulty or non-optimal inputs within the ceLLM framework.
- Gene Expression: This disruption can lead to aberrant gene expression, impaired cellular function, and contribute to the aging process and related diseases.
Integrating Scientific Findings into ceLLM: A Step Forward
The interplay between ECM stiffness and piezoelectric transmembrane proteins exemplifies how the physical and electrical properties of the cellular environment influence gene regulation and cellular health. Integrating these insights into the ceLLM theory underscores the necessity of considering both mechanical and electrical factors in understanding cellular behavior and aging.
Conclusion
The interconnectedness of DNA and cellular structure is pivotal in understanding the ceLLM theory’s depth. As ECM stiffness increases with aging, the resulting mechanical stress on piezoelectric transmembrane proteins disrupts Vmem, leading to faulty gene regulation and cellular dysfunction. This mechanism highlights the importance of maintaining ECM flexibility to preserve bioelectric health, ensure proper gene expression, and promote longevity.
Integrating Gene Expression with Cellular Architecture: The ceLLM Framework
DNA Configuration as the Software Blueprint
Genetic Information
At the heart of every cell lies DNA, the blueprint that contains the instructions for building and maintaining cellular structures through gene expression. These instructions are meticulously transcribed and translated into proteins, which perform a myriad of functions essential for cellular survival, growth, and adaptation. In the ceLLM (Cellular Large Language Model) theory, DNA is not merely a static repository of genetic information but serves as a dynamic software blueprint that orchestrates the cell’s response to internal and external stimuli.
Resonant Fields and Bioelectricity
Within the ceLLM framework, DNA’s resonant properties and bioelectric fields play a crucial role in energy distribution and gene regulation. These resonant fields enable DNA to interact with the cell’s bioelectric environment, ensuring that gene expression is contextually responsive to both cellular and environmental signals. This bioelectric modulation allows for a sophisticated level of regulation, where genes can be activated or suppressed based on the cell’s immediate needs and external conditions, thereby maintaining homeostasis and functional integrity.
Cellular Structures as the Hardware Framework
Cytoskeleton and ECM
The cytoskeleton and the extracellular matrix (ECM) constitute the cell’s physical framework, providing structural support and facilitating the transmission of bioelectric signals. The cytoskeleton, composed of microtubules, actin filaments, and intermediate filaments, maintains the cell’s shape, enables movement, and organizes intracellular components. Meanwhile, the ECM serves as the external scaffold that interacts with the cytoskeleton, influencing cell behavior, signaling, and mechanical stability.
Structural Integration
In the ceLLM theory, the state of the cellular hardware—such as the flexibility of the ECM and the organization of the cytoskeleton—directly influences DNA’s gene expression. This integration ensures that gene expression aligns with the cell’s structural and functional needs. For instance, a flexible ECM allows for dynamic cellular responses and gene regulation, while a rigid ECM may constrain cellular functions and alter gene expression patterns. This structural integration creates a feedback loop where gene expression shapes the cytoskeletal architecture, and the cytoskeleton, in turn, modulates gene expression through bioelectric signaling.
The ceLLM Framework: Integrating Gene Expression with Cellular Architecture
Hardware-Software Synergy
Dual Importance
The ceLLM theory underscores the equally vital roles of both DNA configuration (software) and cellular structural components (hardware) in maintaining cellular identity and functionality. Just as an AI system relies on its hardware and software to operate effectively, cellular systems depend on their structural architecture and genetic configurations to process inputs and generate appropriate outputs.
Interdependence
There exists a profound interdependence between the cytoskeletal network and gene expression within the ceLLM framework. The cytoskeletal network influences gene expression by modulating bioelectric signals, ensuring that cells can respond adaptively to their environment. Conversely, gene expression dictates the assembly and maintenance of the cytoskeletal structures, thereby reinforcing the cell’s structural integrity and functional capabilities. This bidirectional relationship creates a harmonious synergy where hardware and software continuously inform and adjust each other to maintain cellular homeostasis.
Probabilistic Weight Configuration
Weighted Connections
Drawing inspiration from AI systems, the ceLLM theory introduces the concept of weighted connections within gene regulatory networks. Just as AI utilizes weighted connections to process and interpret data, DNA’s gene regulatory networks function as weighted connections that determine the probability distributions for gene expression outcomes. These weights are influenced by various factors, including bioelectric signals, mechanical stresses, and environmental inputs, allowing the cell to fine-tune gene expression in a context-dependent manner.
Adaptive Responses
These weighted connections empower cells to adaptively respond to microenvironmental inputs, such as Vmem changes (membrane voltage alterations) and mechanical stress. By adjusting gene expression patterns based on these inputs, cells can maintain homeostasis and functional integrity. This adaptive capability ensures that cells remain resilient and responsive to fluctuations in their internal and external environments, enhancing their ability to survive and thrive under varying conditions.
Conclusion: The Indivisible Relationship Between DNA and Cellular Structure
The ceLLM framework presents a comprehensive model that emphasizes the indivisible relationship between DNA configuration (software) and cellular structural components (hardware). By integrating the dynamic interactions between the cytoskeleton, ECM, and DNA, ceLLM provides a robust mechanism for understanding how cells maintain their identity, shape, and functionality. This dual emphasis ensures that gene expression is not only dictated by genetic information but is also modulated by the cell’s structural environment, allowing for efficient processing of inputs and generation of appropriate outputs.
As research progresses, validating and refining the ceLLM framework through empirical studies, computational modeling, and interdisciplinary collaboration will be essential. This integrated approach holds the potential to revolutionize our understanding of cellular biology, bioelectric signaling, and gene regulation, paving the way for innovative therapeutic strategies aimed at preserving cellular health and mitigating the effects of aging and disease.